Modeling Muscular Dystrophies
We have developed cell culture substrates that present an engineered cell microenvironment to allow myotubes derived from non-diseased human induced pluripotent stem cells (hiPSCs) to align nearly perpendicular to the patterned grooves/ridges, while myotubes derived from less-affected and severely-affected Duchenne Muscular Dystrophy cells exhibit prominent differences in alignment and orientation. We are using this phenotypic biomarker as a sensitive, low-cost readout in high throughput screening to identify effective drugs or drug delivery methods.
We are also studying the mechanism underlying this phenotype, with an emphasis on elucidating the role of the Dystrophin-Associated-Protein-Complex (DAPC) in mediating the nanotopography-responsive myotube orientation. The DAPC is an important transmembrane protein complex that structurally, mechanically, and functionally links cytoskeleton and the extracellular matrix and serves as both mechanical and signaling hubs in regulating muscle and non-muscle cells.
We have also developed approaches to engineering 3D muscle tissue and functional characterization of the contractile forces generated by muscle constructs. These 3D muscle tissue constructs are also be used in disease modeling.
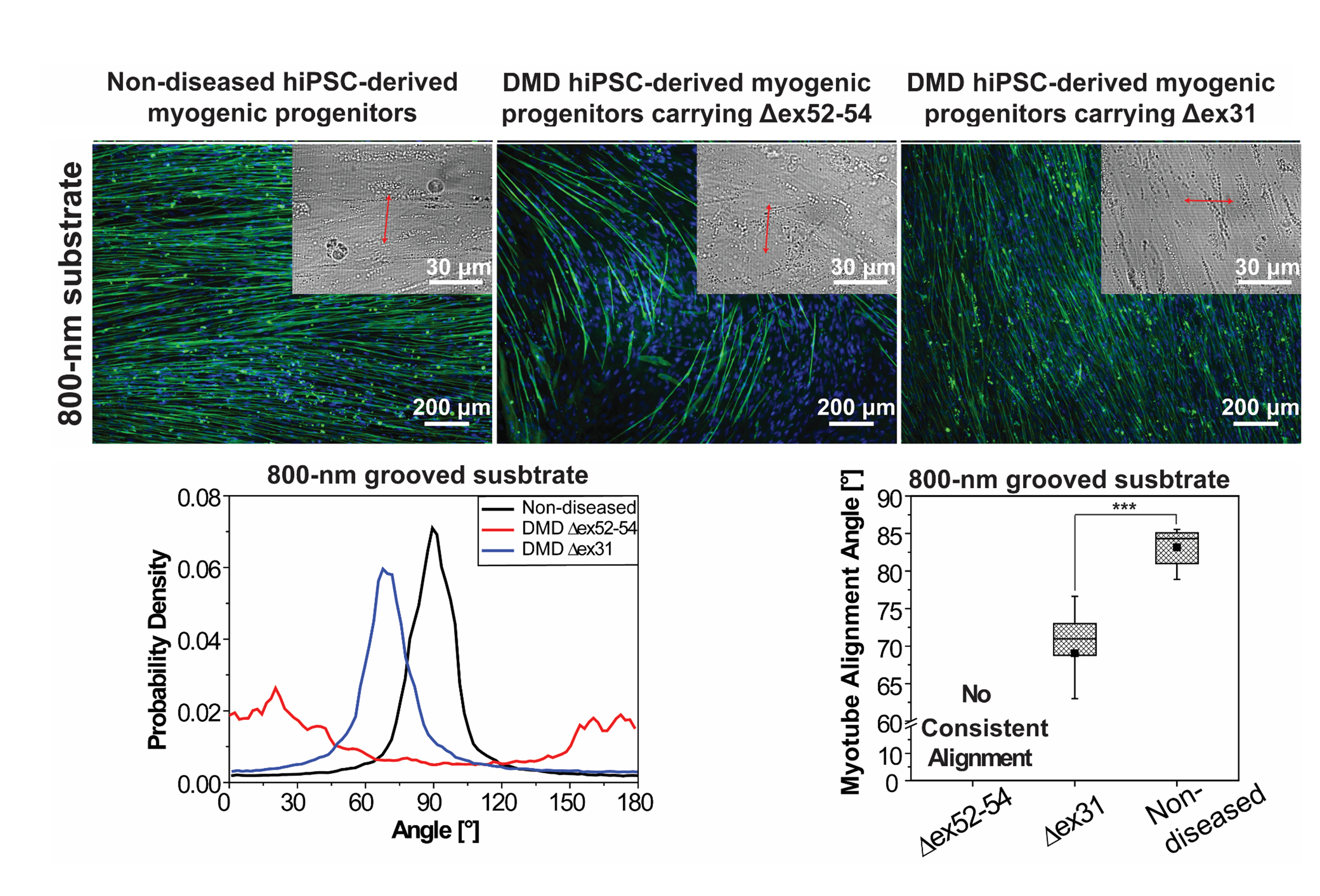
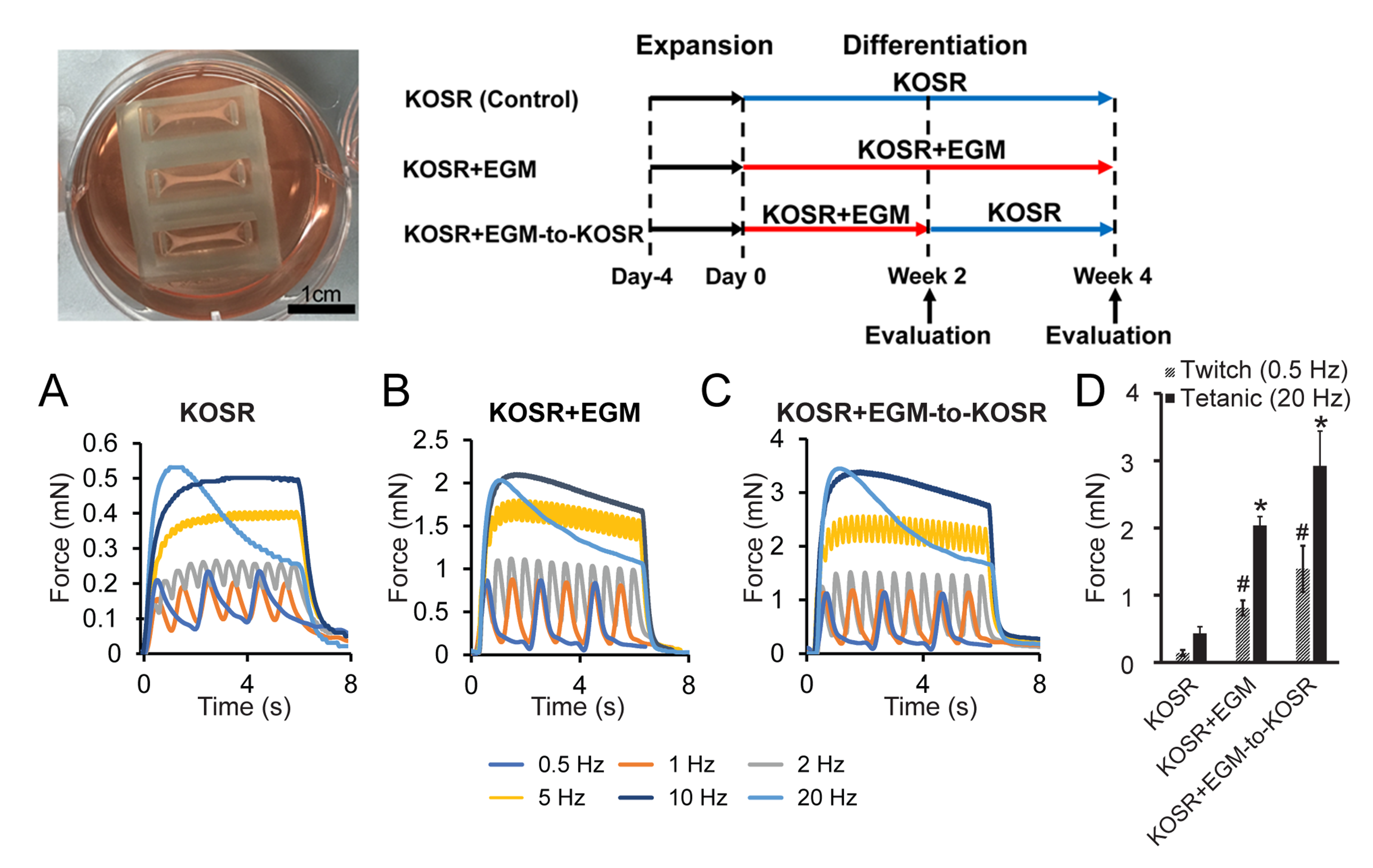
Enhancing Myogenic Transdifferentiation Efficiency Using Engineering Approaches
Human myogenic cells are highly desirable for therapeutic applications and disease modeling. Direct reprogramming from non-muscle somatic cells has become an important strategy to produce abundant, patient-specific, and disease-specific human myogenic cells. As compared to deriving myogenic cells from hiPSCs, direct reprogramming is substantially quicker, and reprogrammed cells avoid the risk of teratoma formation and retain aging-associated and disease-associated epigenetic signatures, which is particularly important for modeling aging-related muscle diseases. Despite these advantages, applications of directly reprogrammed myogenic cells are hampered by low reprogramming efficiency and their immature nature. We are working on enhancing myogenic transdifferentiation efficiency using a variety of engineering approaches.
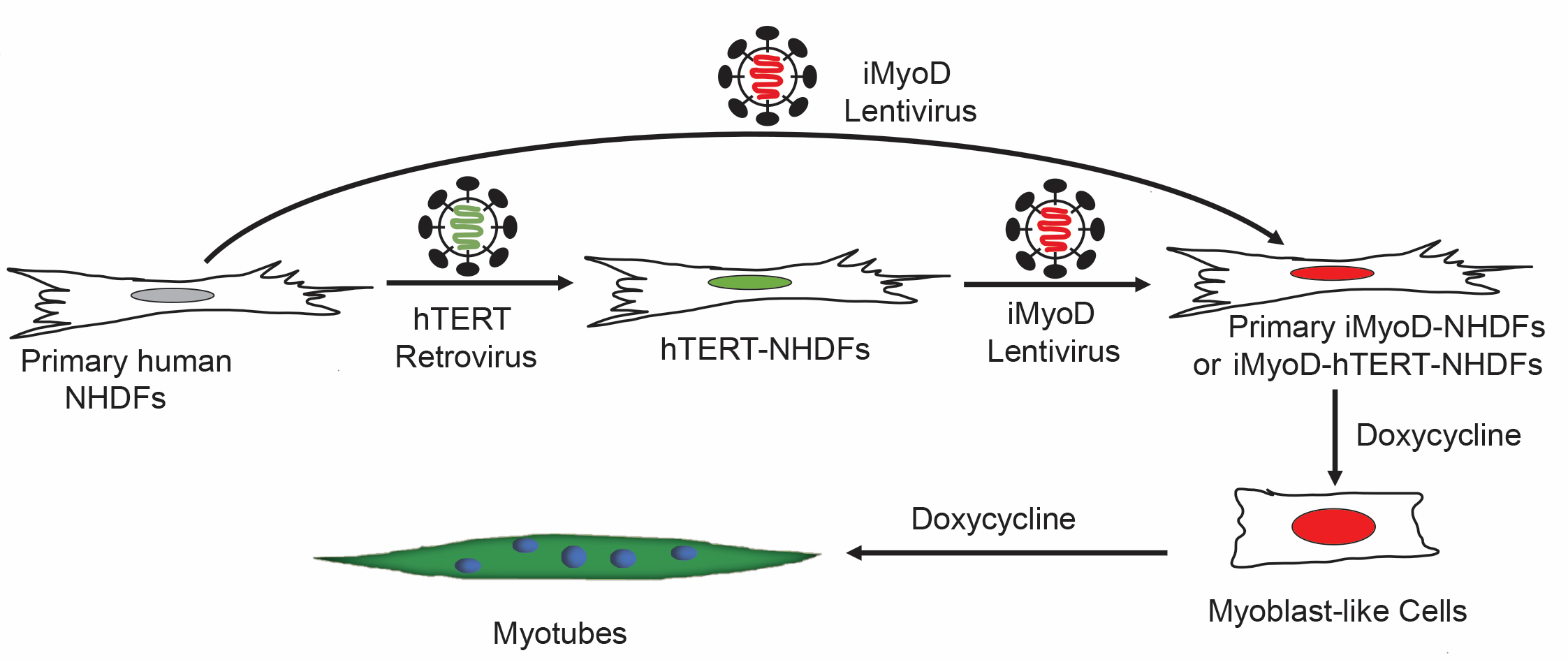
Using Pluripotent Stem Cell (PSC)-Derived Embryo Models to Investigate Chimera Competency Enhancement
Organ transplantation has been limited by the shortage of organ donors. Alternate sources for organ transplantation include humanized xenogeneic organs from large animals such as pigs. Recent advances in gene editing offer the possibility of generating exogenic organs via blastocyst complementation in gene edited animals. However, chimera competency is low when human cells are involved. We are developing PSC-derived embryo models to investigate chimera competency. These embryo models are tractable, scalable, and amenable to genetic and chemical modulations. We use these models to screen conditions for enhancing chimera competency in a high-throughput manner, test hypotheses, and dissect the mechanisms underlying chimera competency enhancement. This is a collaborative project involving several laboratories on campus.
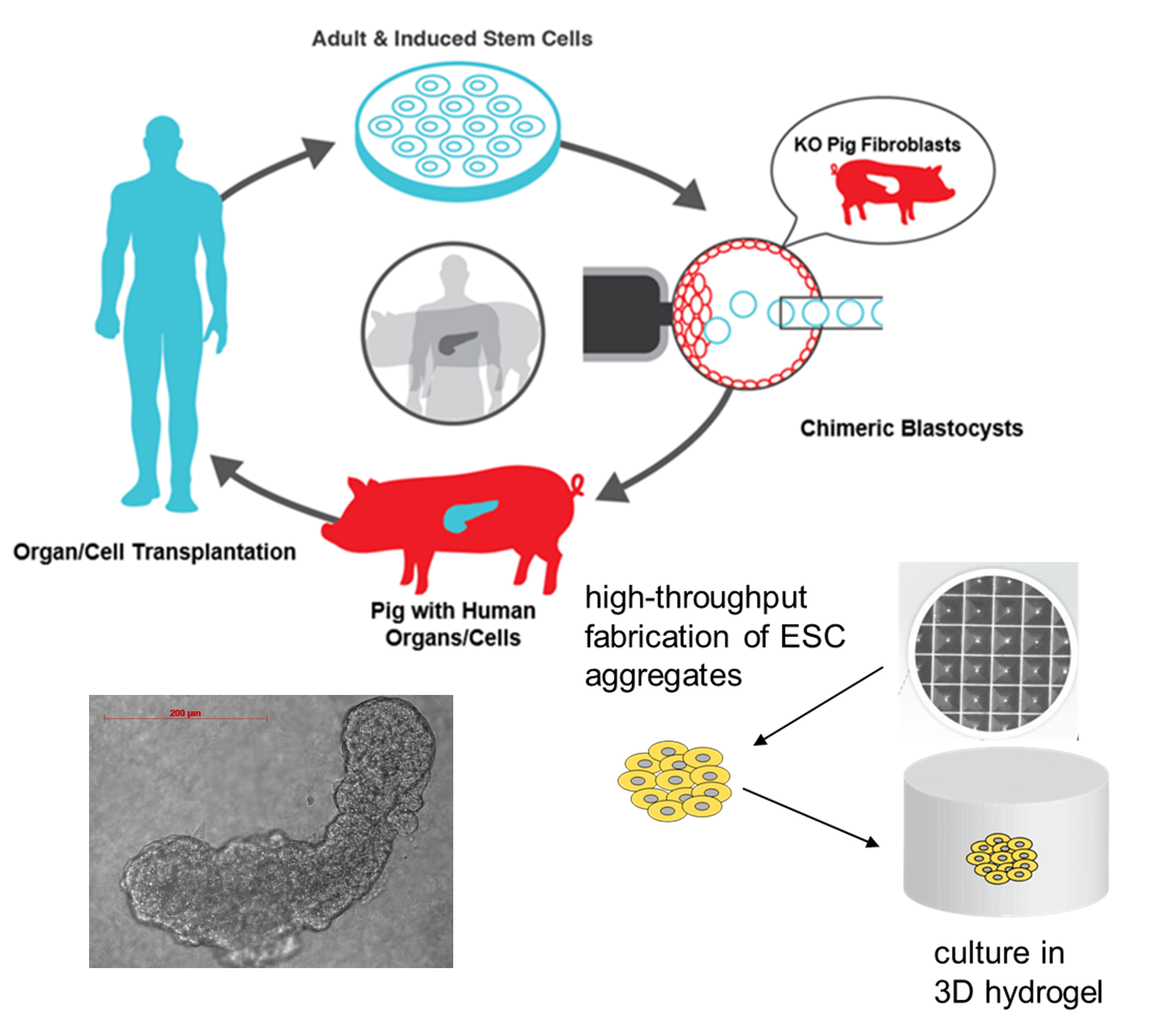
Biodegradable, Thermoplastic, and Implantable Elastomers
Biodegradable and implantable materials having elastomeric properties are highly desirable for many biomedical applications such as medical devices, tissue engineering scaffolds, and drug delivery devices. We have developed biodegradable, implantable, and thermoplastic elastomers that can be processed below 60°C through extrusion, molding, or 3D printing with the possibility of loading and preserving bioactive agents.
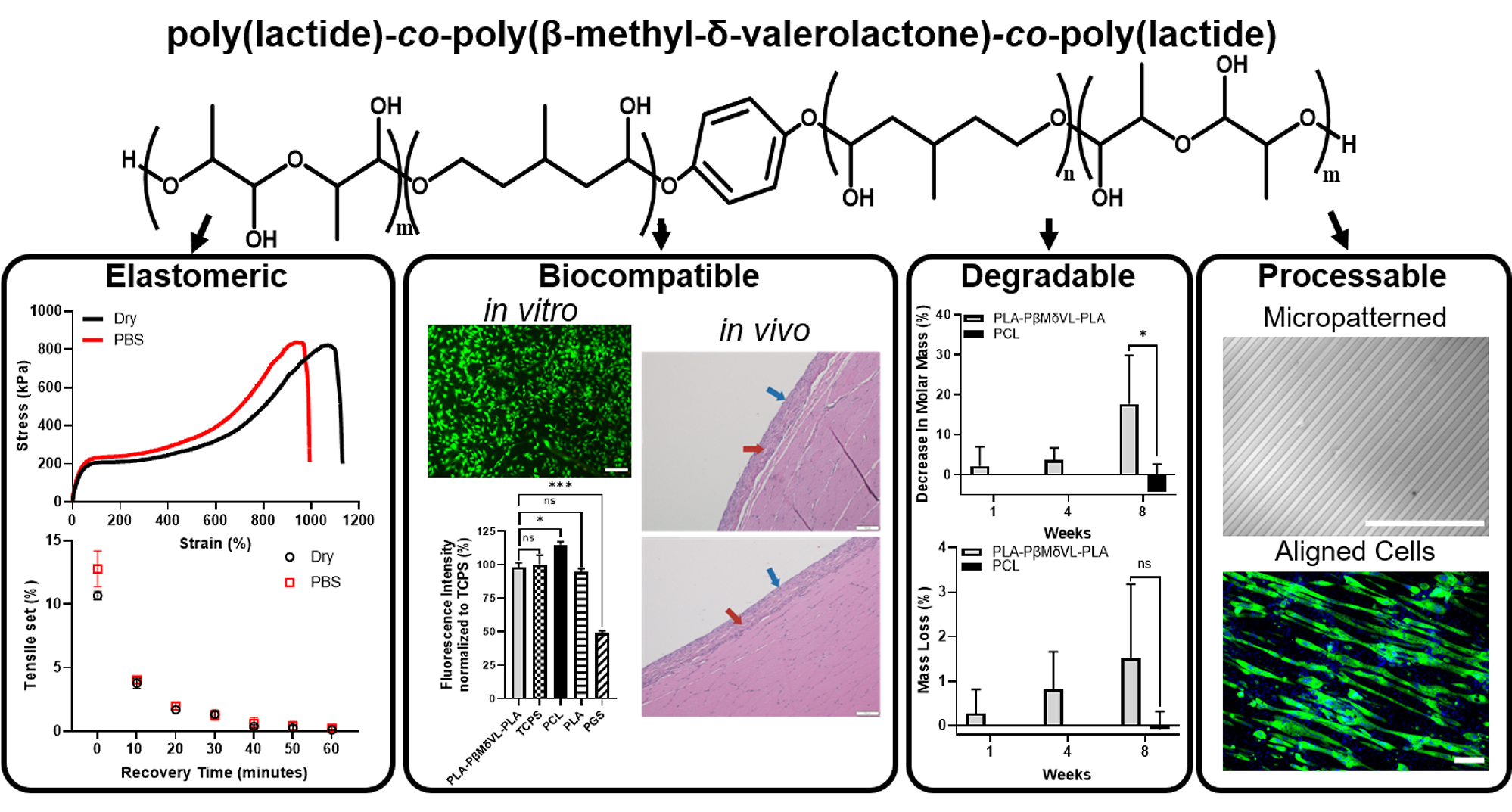
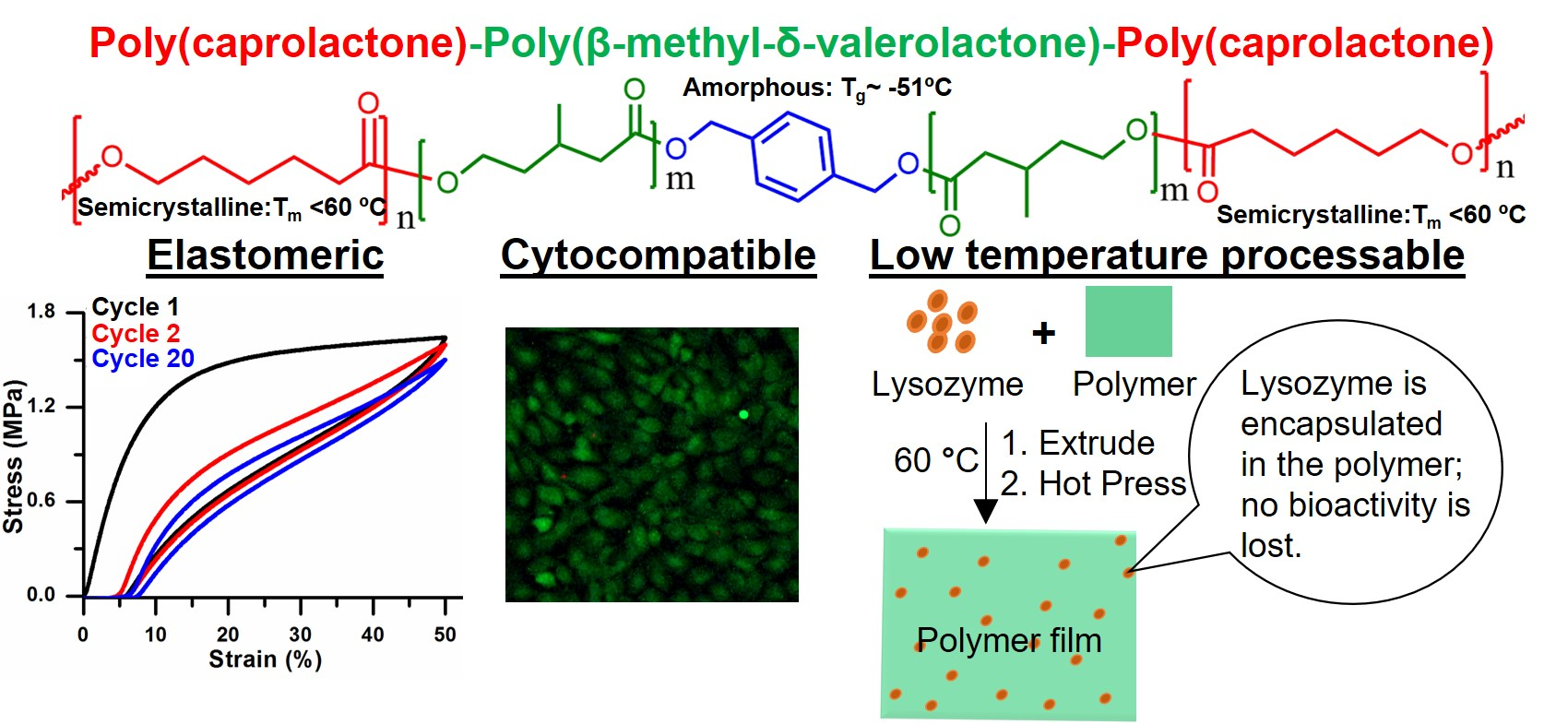
Improved Preservation and Culture of iPSC-Derived Motor Neurons
Human pluripotent stem cell (hPSC) technologies are beginning to realize their potential to improve in vitro modeling of human diseases and provide paradigm changing approaches to treating diseases where current approaches can alleviate symptoms but do not cure the diseases. Neurological disorders of the central nervous system (CNS) such as ALS, Parkinson’s disease, Alzheimer’s and other dementias are key targets for hPSC technology, where human neurons differentiated from patient-derived induced PSCs (iPSCs) can be used to study the underlying mechanisms of disease pathogenesis and test potential therapeutic approaches. However, translation of iPSC-derived CNS neurons is limited by the inability to successfully cryopreserve differentiated, mature neurons and the inability to lift the neural cells from a culture surface and have them re-attach and function. We are developing new improved methods that will significantly advance the utility of culturing and preserving fully differentiated iPSC-CNS neurons for disease modeling, drug screening and therapeutic use. This is a collaborative project involving several laboratories on campus.
Developing Pediatric Medical Devices
We are developing medical devices using various biomaterials to treat pediatric diseases such as Patent Ductus Arteriosus.